The toaster-sized capsule hit our atmosphere at 12 kilometers per second, enduring temperatures of 3,000 degrees Celsius during its fiery descent before deploying a parachute to slow its velocity. It continued to fall until it finally reached terra firma in the Australian outback. Within hours, awaiting teams of scientists located the capsule’s landing site with radar and rushed via helicopter to retrieve it. On board were pieces of an asteroid, the biggest such haul in history, captured millions of kilometers from Earth and returned safely to our planet.
This event, the climax of the Japanese space agency JAXA’s Hayabusa2 mission to the asteroid Ryugu, took place, in local time, on Sunday, December 6, 2020. It marked only the second occasion (the first being its predecessor, the Hayabusa1 mission in 2010) that pieces of an asteroid have been returned to Earth by a spacecraft. With these samples, scientists will be hoping to answer many questions about the history of our solar system, and even our own planet. How old are asteroids like Ryugu, for example? How much water and organic material do they contain? And could they have first brought the raw ingredients for life to Earth billions of years ago?
While most groups of researchers grapple with these outsized questions, one more rarefied cadre will be preoccupied with another, deceptively smaller matter: whether or not Hayabusa2’s samples from Ryugu contain an intriguing ingredient of nearly all known meteorites. So far, no one has been able to explain this ingredient’s origins, yet the ramifications of doing so are tremendous. It may reveal to us not just some nebulous history of the solar system, but also never-before-seen details of the process by which our sun’s retinue of planets formed. In our understanding of how Earth—any planet in the cosmos, in fact—came to be, there may be nothing as important as the mystery of the chondrule.
Chondrules, small seedlike rocks measuring up to just a few millimeters across, are thought to have formed some 4.5 billion years ago, shortly after the birth of our solar system. From there, they became embedded in larger rocks, called chondrites, which make up the majority of the roughly 64,000 meteorites that humans have discovered throughout recorded history. “Chondrules are everywhere,” says Fred Ciesla, a planetary scientist from the University of Chicago. Even so, scientists have been unable to agree on how they formed for almost two centuries. Some think they were byproducts of the planet formation process; others posit they were the seeds of planet formation itself. Either way, the menu of chondrule formation scenarios is vast, ranging from lightning-fused dust to colliding chunks of protoplanets to giant gas-heating shock waves rippling through the primordial cloud of material that surrounded our newborn sun.
Understanding chondrule formation could, in other words, reveal our solar system’s earliest moments. And now, with fresh or prospective results from missions like Hayabusa2 as well as other avenues of research, chondrule-obsessed scientists are on the cusp of answering the centuries-old question of where they—and perhaps we—came from. “They are stained glass windows to the earliest time period of the solar system,” says Harold Connolly, a cosmochemist and chondrule expert from Rowan University in New Jersey. “They are witnesses to processes that operated in the early solar system. The question is, what did they witness?”
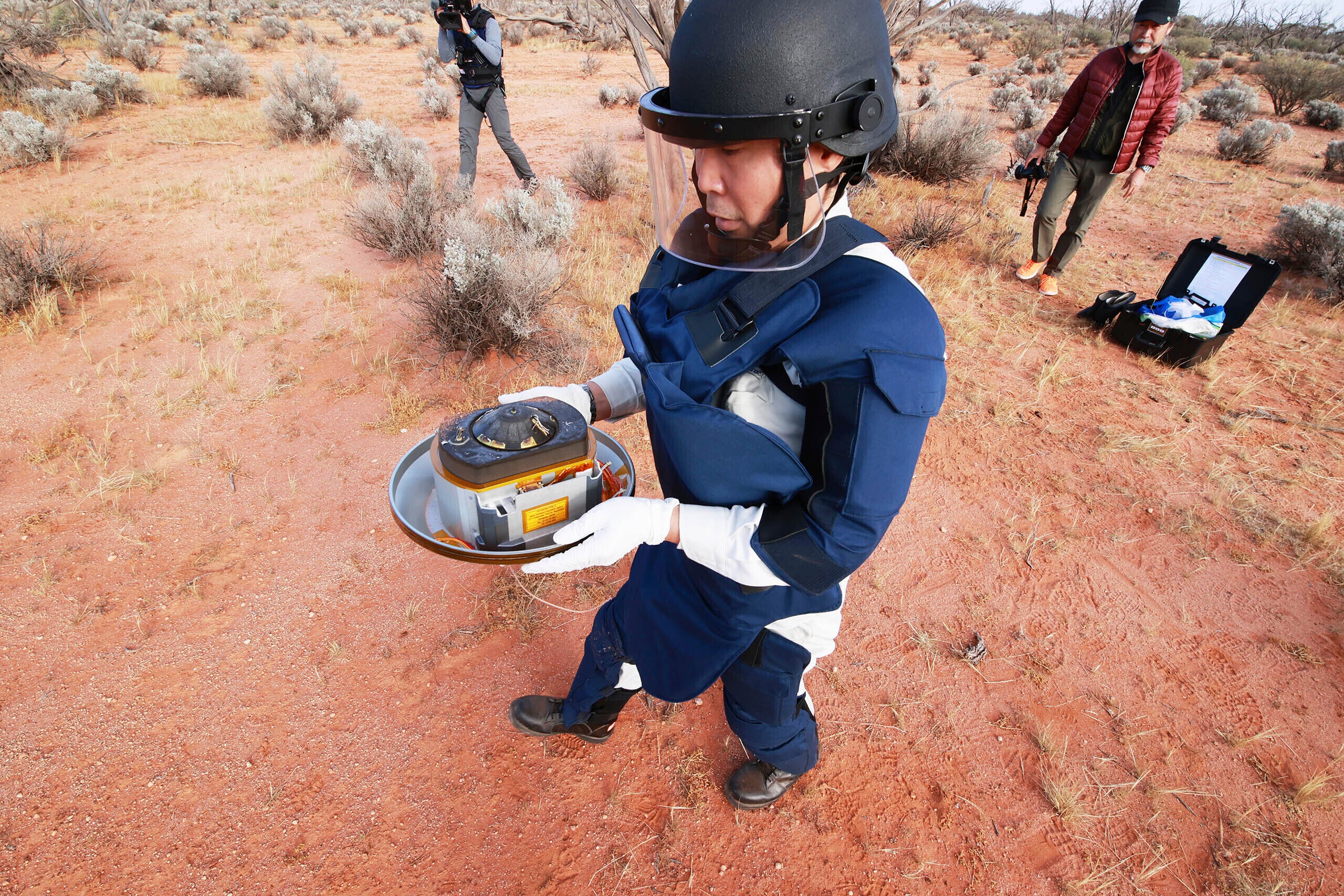
DROPLETS OF FIRE
In 1802, the British chemist Edward Howard was one of the first scientists to recognize chondrules as “rounded globules” in meteorites. Their name, given later by the German mineralogist Gustav Rose and Austrian mineralogist Gustav Tschermak, originates from the Greek chóndros (“grains”) and the German kleine kugeln (“small balls”). In 1863, the British scientist Henry Sorby would characterize them in greater detail, describing chondrules as “droplets of fiery rain,” molten globules that condensed around the sun, although, then as now, the exact mechanism of their formation was unclear.
The broad outlines of our solar system’s formation are far less contentious, incrementally assembled via careful observation and modeling. This creation story begins more than 4.5 billion years ago, when dust and gas from a giant molecular cloud gravitationally collapsed to create a protostar that would become our sun, surrounded by a spinning disk of gas and dust. Within this disk, a mix of gravity, aerodynamics and electrostatic force caused grains of dust to stick together, building larger and larger agglomerations—such as planetesimals, the kilometer-scale building blocks of planets—which ultimately formed the planets themselves within perhaps just a few million years. These worlds gradually settled into familiar forms and orbits, giving rise to the solar system we know today. The delicate intricacies of how we reached this point are where things get mysterious, however. Chondrules concern the opening chapters of the story, the leap from dust to planetesimals. How do you get from microscopic motes to entire worlds thousands of kilometers across?
Essentially rocks within rocks, chondrules appear as rounded flecks in chondritic meteorites. Some are visible to the naked eye, whereas others can only be seen under a microscope. They would have solidified in the cold expanse of space after their unknown formation process, beginning their lives as molten droplets before coalescing into chondrites. It is difficult to overstate just how abundant chondrules are: Despite the fact that none are known to have survived the process of incorporation into planets, they are very common off-world, often constituting the bulk of material within chondrite meteorites. Some chondrites are so chondrule-packed they look almost like a conglomeration of beads.
Made of minerals such as olivine and pyroxene, and sometimes glass, chondrules themselves come in a variety of shapes, sizes and compositions—often containing a glittering array of crystals. Scientists can date their formation to a window of a few million years 4.567 billion years ago by measuring the abundance of an isotope of aluminum they contain called aluminum-26. “This is a short-lived nuclide that only exists in the early solar system,” says Noriko Kita, an expert in meteorite aging from the University of Wisconsin-Madison. That advanced vintage makes chondrules the second-oldest recognizable objects in our solar system, after calcium-aluminum-rich inclusions (CAIs), specks of white in meteorites that are thought to have formed one to three million years earlier by condensing out of the gas that surrounded our young sun.
There are many classes of chondrites. Ordinary chondrites, for example, are chock full of chondrules and account for more than nine out of every 10 chondrule-containing space rocks. Carbonaceous chondrites, which account for about 4 percent of all chondrites, have a high abundance of carbon and are very similar in composition to the sun’s surface, suggesting they formed in the inner solar system. A subgroup called CI chondrites have no visible chondrules, having likely been altered by water. And CB chondrites hold a particularly unique accolade, being the only type of chondrite for which there is near-universal agreement on how they formed. “These are a group that we think formed during one giant impact,” says Sara Russell, a planetary scientist from the Natural History Museum in London. That pinned-down provenance makes them “kind of magical.”
Because apart from this one anomaly, the exact formation process for chondrules and chondrites remains a mystery—but not for any lack of trying. For decades scientists have devised and tested myriad different formation models, but a consensus has remained elusive. “It’s frustrating, but also it’s kind of fun that we don’t know,” says Russell. “They’re obviously telling us about some ubiquitous, superimportant process about how our solar system formed. We just have to work out what that is.” Figuring out the mystery, however, has not always been smooth sailing.
AN IMPOSSIBLE PROBLEM
In 2000, at the Lunar and Planetary Science Conference in Houston, a stunned audience watched as John Wood—one of the most revered scientists studying chondrules—appeared to admit defeat in understanding their origin. Like many before him, Wood had become fascinating by chondrules when he first laid eyes on them. “[These] little balls of stone were so charming and interesting and mysterious that I just got seduced by them,” he says. But he was frustrated at the lack of progress that had been made. “We still don’t understand what the meteorites are telling us, and sometimes I wonder if we ever will,” he later wrote in a summary of his speech. A few years later, facing a lack of funding, he opted to retire, turning his attentions to oil painting and spending time with his wife. “I quit science cold turkey,” he says.
The speech was a shock to many. “He basically said he’d wasted his entire life working on chondrules, because it was an impossible problem to solve,” says Conel Alexander, a cosmochemist from the Carnegie Institution for Science in Washington, D.C. “That got a lot of people pretty upset.” Larry Nittler, also a cosmochemist from Carnegie who was in the audience, says he “actually stood up” to defend chondrule research. “I said, ‘I’m still excited about these incredible rocks from space,’” he recalls. “I don’t think I’ve gotten as much attention or praise for anything I’ve done in my career. The whole room erupted in applause.”
Yet one could be forgiven for pessimistically siding with Wood. After all, space scientists have managed to definitively solve a wealth of seemingly intractable mysteries—teasing apart the very first moments of the universe’s existence, discovering worlds around other stars, observing gravitational waves and even capturing images of a black hole. Against such magnificent achievements, the stubborn enigma of the lowly chondrule seems to shrink even smaller than its already niche status. Today there are, the joke goes, as many theories about chondrule formation as there are chondrule scientists themselves—with the acerbic addendum that tomorrow there will inevitably be even more. The problem of chondrules has from the start been intergenerational, inspiring one cohort after another to try their hand at tackling the issue, with varying success.
The main problem is finding a model that can explain all the different, diverse properties of chondrules. “There are no models that tick all the boxes,” says Alexander. To make chondrules, dust must have been heated to temperatures of up to 2,000 degrees Celsius by some process in the early solar system, before rapidly cooling over just days or even hours. This process, whatever it was, likely occurred throughout the solar system, in order to account for the large abundance of chondrules found in chondrites on Earth. And the chondrules must also have drifted for a time through the dusty environs around our young sun, to account for the telltale rims of accumulated dust that encase their centers.
Most chondrule scientists fall into one of two camps. The first believes that chondrules were among the first solid objects to appear in the solar system, forming directly from the solar nebula—the cloud of dust and gas that surrounded our young sun. This would make chondrules a key stepping-stone from miniscule dust to larger kilometer-sized planetesimals. The second camp believes that chondrules were not among the first solids to form, but actually arose after planetesimals—perhaps even after the planets themselves—being instead a by-product of the planet-formation process rather than being actively part of it.
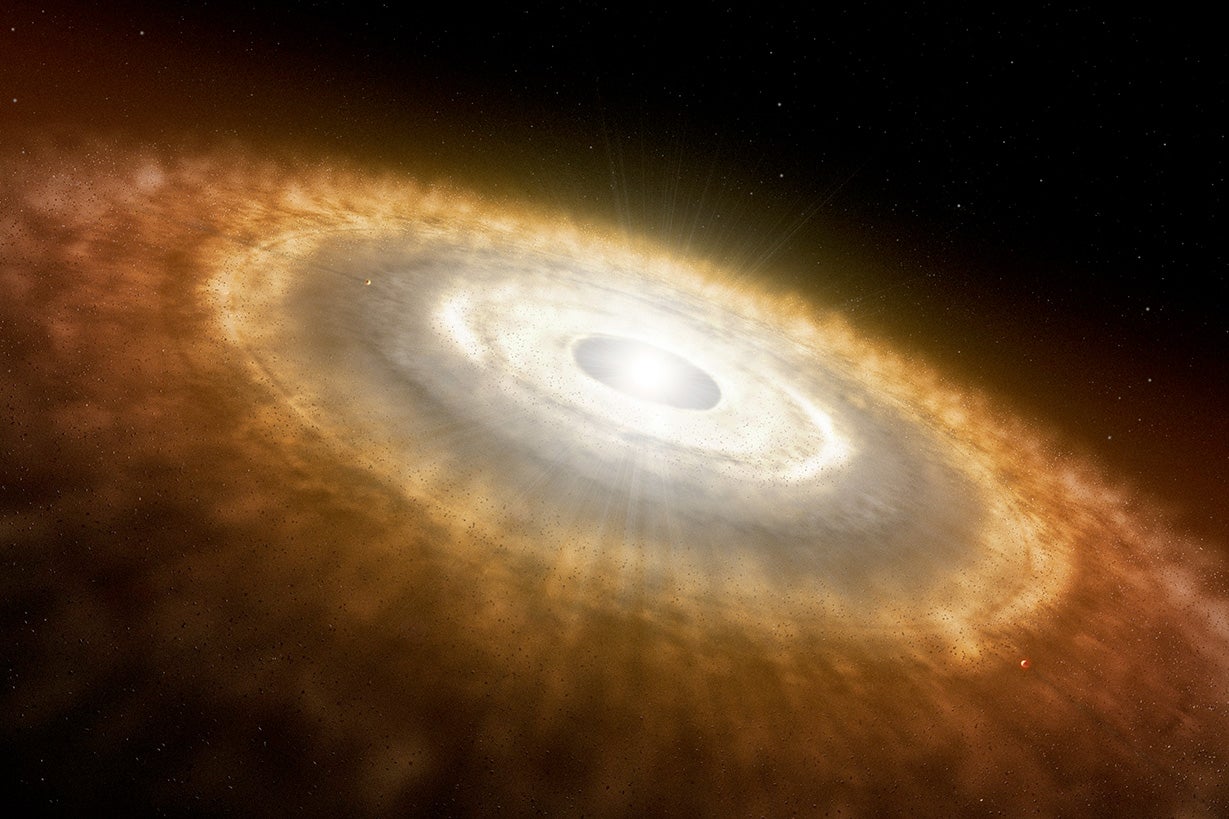
Within the first camp, one idea is that gravitational instabilities in the disk of dust and gas around our sun resulted in “shock fronts” that fused some of the dust into chondrules. “If you look at a picture of a galaxy you see spiral arms spiraling round, and the same thing would have happened in the protoplanetary disk,” says Rhian Jones, a cosmochemist at the University of Manchester in the U.K. “And you can produce shock fronts associated with those density differences between the clumpy arms and the gas.”
The more radical nebula lightning model, meanwhile, suggests friction between dust and gas particles around the sun sparked immense discharges of electricity that fused this dust into chondrules, although it’s not entirely clear how such lightning would be produced. Other models in this camp include magnetohydrodynamics, where huge magnetic fields would trap sheets of electric current to create hot spots tens or hundreds of thousands of kilometers across that melt dust grains to churn out chondrules.
In the other camp, which proposes chondrule formation was post-planetesimal, one of the more prominent models is called impact jetting. Here, planetesimals would collide at high velocities, creating the necessary heat to produce chondrules. “It essentially squirts out some molten material that could break up into droplets,” says Brandon Johnson, a planetary scientist from Purdue University in Indiana. A variant of this, called splashing, would have involved collisions between molten objects at lower velocities, releasing droplets into space that solidified into chondrules.
The nebula shock model, meanwhile, posits that Mars-sized planetary embryos moving through the nebula could act like boats sailing through water, fusing dust into chondrules. “As it’s moving through the gas at supersonic speeds, it drives a bow shock,” says Steven Desch, an astrophysicist from Arizona State University. “The chondrule precursors, this dust, is heated by entering this compressed hot gas and is processed by it.”
Other ideas include radiative heating, a relatively new idea that suggests planetesimals flying low over molten bodies could have been roasted and then cooled to produce chondrules. Rocks that underwent this natural “heat treatment” process would be sturdier, and more likely to survive the passage through Earth’s atmosphere, handily explaining why most of the meteorites we find are chondrites. “The meteorites got hardened, and Earth’s atmosphere is a filter that is weighted towards really dense and hard stuff,” says astronomer William Herbst from Wesleyan University in Connecticut, one of the researchers behind the idea.
Against this rising theoretical tide, some wilder notions have already been ruled out. Events from outside the solar system such as gamma-ray bursts—enormously energetic explosions from sources such as merging neutron stars or black holes—were once considered a possibility, but now seem implausible owing to the great distances involved. Even so, many models still remain, complicated by the fact that chondrules are not really predicted by planet formation at all. “We can build a story about how planets form without ever invoking chondrule formation,” says Ciesla. “It’s obvious that there’s a piece of the story that we’re missing.”
Narrowing down which of the remaining theories is correct is hard, and arguments can get heated. “The best way to make friends and enemies in meteoritics is to publish another chondrule-forming model,” says Connolly. At stake is what role chondrules played in our solar system. If they were among the first solids to form, then some inescapable process took place around our young sun that could explain how planet formation begins around most any star. But if not, are they less vital to the process than once thought?
“I’m putting my money on collisions right now,” says planetary scientist Eugene Chiang from the University of California, Berkeley. “And to be totally up front about it, that makes [them] a little less interesting. Because if you’re interested in planet formation, it means the chondrules are not the most primitive objects. They’re secondary products.”
COOKING UP CHONDRULES
Most of our ideas on chondrule formation come from modeling of the early solar system and performing experiments on Earth to replicate different formation methods. Meteorite scientist Aimee Smith from the University of Manchester and her colleagues are one of several teams around the world that perform such experiments, mixing chemicals into a powder to resemble different types of known chondrule compositions. Then they place the powder in a furnace and heat it to extremely high temperatures for anywhere from hours to days, before cooling it to mimic different formation models. “If we get ones that look similar to the natural chondrules that we’ve studied, then we have a better of idea of how they formed,” Smith says.
Experiments like these are crucial for understanding chondrules in concert with modeling of the solar system. “The experiments are just defining the conditions for chondrules,” says Jones, Smith’s supervisor. “But models are trying to come up with scenarios in which those conditions are satisfied.” And in our solar system, such modeling is starting to paint a new picture of its earliest moments.
Recent work on measurements of isotope ratios in meteorites suggest two different reservoirs of chondrites formed early on, one in the inner solar system and one in the outer solar system, where chondrules may have been produced separately. These separate populations would have mixed together after Jupiter, having initially formed more than twice as close to the sun, migrated out to its present position, an idea called the grand tack hypothesis. If true, this would suggest that the story of our solar system’s turbulent history is stored within chondrules themselves, offering yet another reason to lavish them with careful attention.
Elsewhere, observations of other solar systems—in particular, protoplanetary disks of dust and gas around young stars—are yielding useful information about possible scenarios for chondrule creation. In 2014, astrophysicist Huan Meng from the University of Arizona and colleagues saw evidence for a potential protoplanetary smash-up around a star called NGC-2547 ID8 more than 1,000 light-years from Earth, in the form of a flash of infrared light. While not definitively linked to the formation of chondrules, the observation at least showed that suitably energetic collisions to make them do seem to occur in young systems. “Before [our] paper, we didn’t have any direct hard evidence of any extrasolar planetary impacts,” says Meng.
In the future, we should also be able to better probe the distribution of dust around young stars with higher resolution images, letting us possibly refine some models of chondrule formation. “With improvements in techniques and telescopes, now we can start to see the dust production around young stars,” says Yves Marrocchi, a planetary scientist from the French National Center for Scientific Research. “Maybe in the near future we can see the formation [process] of chondrules.” Such telescopes may include NASA’s much-delayed James Webb Space Telescope, scheduled to launch in October 2021, which could provide some intriguing answers in the infrared.
If chondrules are among the first solids to form around stars, they could be crucial catalysts for subsequent planet formation, in particular the jump from dust-sized to kilometer-sized objects. “There’s a gap when you need multi-meter to kilometer size objects to actually form the rocky parent bodies of planets,” says Connolly. “What happens in that gap becomes really important.” And perhaps chondrules are even more essential; one model of planet formation, pebble accretion, posits that larger bodies swept up pebblelike dust in order to grow into planets. Could those pebbles in fact be chondrules? “We don’t know if they’re the same,” says André Izidoro, a planetary scientist from Rice University in Texas. Finding out would likely require “a big sample of an asteroid,” he says. And as it happens, we just got one.
“NO SINGLE SENTENCE ANSWER”
Preliminary estimates suggest Hayabusa2 only managed to bring back about a gram of material from asteroid Ryugu, but according to JAXA’s sample analysis team lead Shogo Tachibana, that should be enough to see if chondrules are present. He and his team will begin studying the samples in earnest next year, after they are transported from Australia back to Japan. What they will find is anyone’s guess. “We don’t know if chondrules in Ryugu are different from other types of chondrules in other chondrites,” says Tachibana. Ryugu appears to be similar to Earth’s carbonaceous chondrites, so most experts expect chondrules to be present in the samples, although none know whether they will resemble those already in collections or will be like nothing ever seen before.
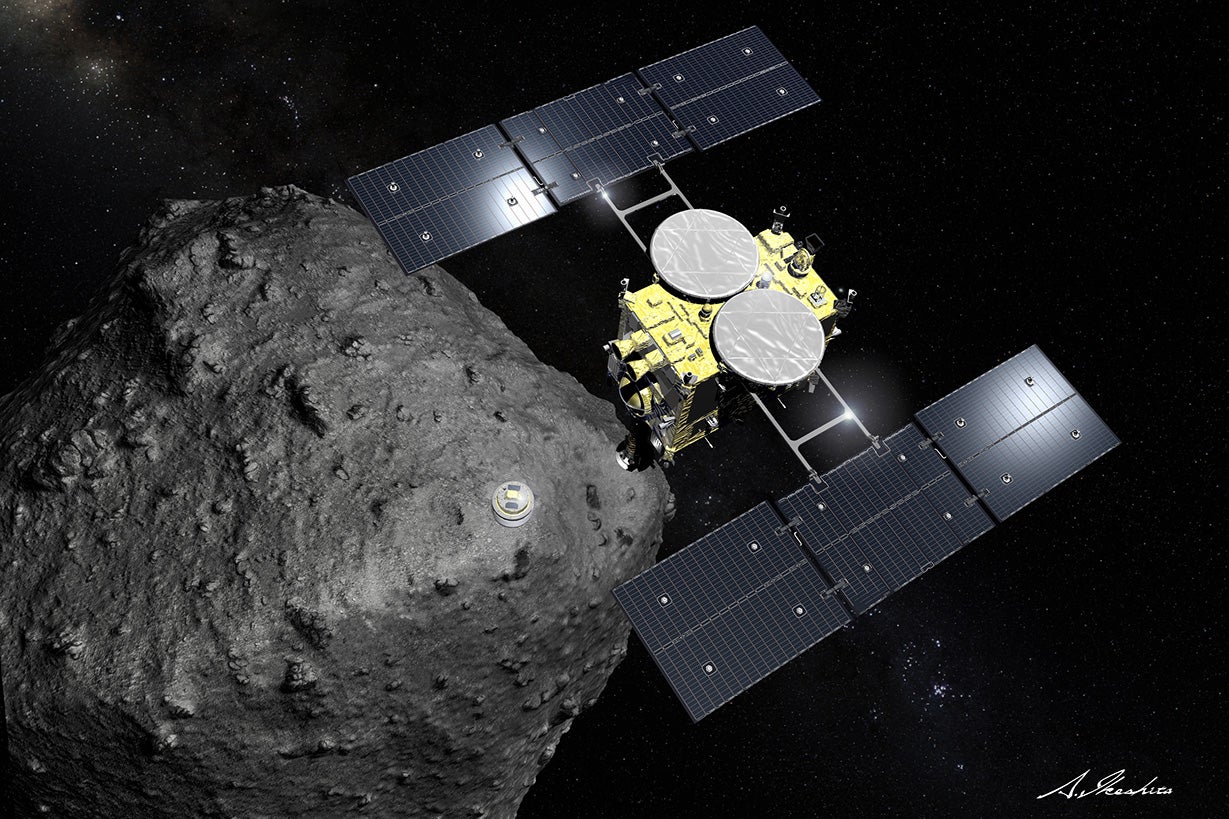
It could be, however, that Hayabusa’s samples prove to be chondrule-free. “I think that would be shocking to the chondrule community,” says Herbst, overturning any already precarious consensus. “If there were no chondrules, and it looked like there had never been chondrules in them, then maybe chondrule formation is not such a ubiquitous process,” says Russell.
Early results from a lander called MASCOT, deployed by Hayabusa2 onto Ryugu in October 2018, have already tantalized scientists. Images from the lander showed many white markings on the surface, which may have been CAIs, but also could have been chondrules. “We were surprised that we really could see [the markings] and that there were so many of them,” says Ralf Jaumann, head of MASCOT’s science team at the German Aerospace Center, or DLR, Germany’s space agency. Only chemical studies of the samples back on Earth will reveal the markings’ nature with certainty.
If chondrules are present, and similar to existing chondrules we know of, scientists will be able to link these to a specific planetesimal—namely Ryugu—to pinpoint the location, time and perhaps even conditions in which they formed in the solar system. If new types of chondrules are found in the samples, however, that could give us a fresh perspective on the larger problem of origins—and scientists like Connolly favor such a scenario. “I certainly hope there’s a few surprises and we find objects we didn’t expect,” he says. And even if chondrules are not present, it could simply suggest Ryugu has been altered by water in its past, erasing any evidence of its chondrules, as with CI chondrites found on Earth.
Hayabusa2 is not the only sample return mission with extraterrestrial gifts in store for chondrule scientists. NASA’s ORISIS-REx spacecraft is scheduled to return to Earth in September 2023 with recently acquired samples of another asteroid, called Bennu, that are expected to be chondrule-rich. “It would be really disappointing if we didn’t find chondrules in the material,” says Connolly, who is also part of the OSIRIS-Rex team. “I’m looking forward to finding chondrules that I know and chondrules that I don’t know.”
These two missions alone are not expected to solve the issue of chondrule formation, but they offer vital insights into the broader chondrule problem, with a major question yet to be answered: Were chondrules, these tiny molten droplets that solidified in space, the seeds that led to the formation of planets like Earth? Or were they the by-products of a violent formation process as hunks of rock smashed together? “Now more and more people are talking about a planetesimal source,” says Myriam Telus, a planetary scientist from the University of California, Santa Cruz. “I do think coupling of the modeling and meteorite observations will get us to the answer.”
For some, the solution will be anything but simple, and perhaps more than one theory is correct. “I do not think it is a single sentence answer,” says planetary scientist Sarah Stewart from the University of California, Davis. “There were probably many droplets being made in different ways.” Russell agrees. “My favorite theory is that everyone’s right,” she says. “All of these processes happened somewhere in the solar system. There were shock waves, there were impacts, there were bow waves, there was lightning. I think these things all happened, and they all formed chondrulelike objects.”
What is clear is that across two centuries, these little droplets of fire have equally bemused and amazed countless curious scientists, and continue to fascinate. Despite the frustrating lack of certainty about their origins and significance, the desire to know their secrets is going nowhere. “If I had to do it all over again, I would have made the same attempt,” says Wood. And to anyone following in his footsteps? “I would wish them good luck,” he says.
"creation" - Google News
December 08, 2020 at 06:45PM
https://ift.tt/3gtlwtN
Asteroid Dust from Hayabusa2 Could Solve a Mystery of Planet Creation - Scientific American
"creation" - Google News
https://ift.tt/39MUE4f
https://ift.tt/3bZVhYX
Bagikan Berita Ini
0 Response to "Asteroid Dust from Hayabusa2 Could Solve a Mystery of Planet Creation - Scientific American"
Post a Comment